OR/15/001 Guidelines for use
Jordan, J J, Grebby, S, Dijkstra, T, Dashwood, C and Cigna, F. 2015. Risk information services for Disaster Risk Management (DRM) in the Caribbean. British Geological Survey Internal Report, OR/15/001. |
The land use/land cover maps produced under Service 1 provide detailed information on the spatial distribution of 14–15 different classes in St. Lucia, Grenada, and St. Vincent and the Grenadines. With a spatial resolution of 2m, these maps are equivalent to a map scale of 1:10 000. The accompanying vector layers of water bodies, rivers and streams and the basic road network were also mapped at 1:10 000-scale. Accordingly, the intended scale for use of these data is 1:10 000, and so may not be representative at finer scales. While the land use/land cover maps provide 100% coverage of the AOIs, some of the vector layers may appear somewhat fragmented due to cloud and shadow obscuring the ground in the Pleiades satellite imagery. It is also worth noting that although the land use/land cover maps have high overall accuracies, some confusion still persists between classes that are inherently similar. The predominant source of this confusion is due to overlap and/or similarity in the types of trees present in different types of forest. Enhanced discrimination between these types of forest could be achieved with the aid of satellite imagery acquired at periods reflecting different conditions (i.e. leaf-on and leaf off). Overall, the new land use/land cover maps represent a considerable improvement on the existing 30m maps.
Within the context of this project, the land use/land cover maps produced here can be used to determine whether there is a spatial correlation with landslide occurrences documented in Service 2. Such analysis is useful in establishing whether specific land use/land cover types are more prone to landslide events, and can thus be used as input, alongside the DEMs (also generated in Service 2) to derive landslide susceptibility maps for St. Lucia and Grenada.
Additionally, the DEMs generated in Service 2 can also be used in conjunction with the water surface features mapped under Service 1 to model the flood risk in both St. Lucia and Grenada. Furthermore, the land use/land cover maps could be readily turned into impervious layers, which can also be incorporated in flood risk analysis. The 20m and 30m DEMs produced for Service 3 can also be used to model flood risk in Belize. However, prior to use in flood risk modelling, it is advised that the DEMs generated here are checked to ensure they are hydrologically correct.
Beyond the scope of this project, the land use/land cover information can be used for a wide spectrum of uses. For example, the maps could be used for planning purposes, asset management and in developing forestry management strategies. The data can also be used to monitor change over time. Some broader applications of the DEMs could include forestry management, the planning of new transport infrastructure (i.e. roads and railways), and natural resource exploration.
Landslide inventories for St Lucia and Grenada contain polygons that represent the maximum extent of events mapped during the period 2010–2014. Each polygon is attributed with landslide type, morphology, confidence level of the mapped outline and a statement of activity for each of the five years in the sequence 2010–2014. The inventory thus provides a clear indication of landscape response to trigger events. Interpretation of satellite images requires well-trained operators with a good understanding of mass movement processes and local conditions.
Information on trigger event response, spatial distribution, magnitude-frequency, type of movement, etc. can be very valuable for the development of derived products such as landslide susceptibility maps and landslide risk assessments. It is therefore envisaged that this product will provide context to future studies of landslide hazard (such as e.g. CDB and CDERA 2006[1], ECLAC 2011[2]) and landslide risk reduction (e.g. CHARIM — van Westen, 2014[3]; MoSSaiC — Holcombe and Anderson, 2010[4]; Anderson and Holcombe, 2013[5]).
The morphology of the landscape constrains the dimensions of landslides that are encountered with many events occurring on short, steep slopes. A substantial number of landslides have dimensions smaller than 1000 m2 (the effective minimum size for an event that can be mapped on a 1:20 000 scale) and therefore the inventories were established on a scale of 1:10 000.
The latest satellite images provide substantial improvements in resolution that enable very detailed interpretations of the landscape to take place. There is therefore scope to map events smaller than the minimum size captured by the current inventory. It is also good practice to populate each polygon with additional information that can be stored in a relational database for further analysis (i.e. through connecting landslide polygons to landslide point data; see Gibson et al. 2013; Figure 44, and listed in http://nora.nerc.ac.uk/18890/). This will require substantial further work, but will result in an invaluable tool for future landslide hazard and risk assessments.
The current multi-temporal sequence can be extended backwards by incorporating older satellite images thus providing incremental quality enhancements of the database. Regular updates of the inventory (at least annually, but inventory establishment immediately following a trigger event should be a priority) will also provide important value. As the database updates continue, this can be of great value to evaluate the vulnerability of the landscape and estimate the potential consequences of a trigger event (see e.g. Foster et al. 2012[6]; Gibson et al. 2013[7]; Pennington et al. 2014[8]).
The susceptibility of the terrain to generate landslides is not static and therefore trigger magnitudes capable of generating widespread disruption are also variable. For example, in periods shortly following a major disrupting event, much material is still in a critical condition and a relatively small trigger can result in large (re-) mobilisation of hillslope materials. If the landscape has had some time to regenerate a vegetation cover and re-establish a degree of stability fewer events will be active and visible on satellite images. Event capture using satellite images is therefore dependent upon the time difference between a trigger event and the capture of its aftermath by a satellite image. Using a multi-temporal image stack enhances the likelihood that events are being captured and a cumulative inventory will increasingly represent the distribution of unstable terrain.
The landslide inventory database can be interrogated in terms of the annual distribution of active and inactive (but still recognisable) events and this is of great potential value for subsequent analysis, for example in terms of event-response signatures, trigger threshold magnitudes and non-static hazard mapping. There is great value in maintaining and updating a multi-temporal landslide event database at least annually.
The cumulative inventory represents the outlines and associated metadata (Table 3) of landslide polygons for the period 2010–2014. Within the constraints of this project only a simple attribution of landslide type and morphology (undifferentiated) was possible, but enhancement of polygon outlines and further distinctions (for example reflecting better spatial constraints of areas of activity as a landslide becomes increasingly less active) are feasible if more time is available for interpretation of the imagery. The additional information could be maintained in a (relational) database with polygons representing the development of the landslide over time and tables identifying the attributes of the landslide at each point in time. Most features are recognised by bare earth and represent the remains of translational or flow type movements. Few polygons contain features of deep-seated rotational movement where it is theoretically possible to max scar, slide body, etc (Figure 20).
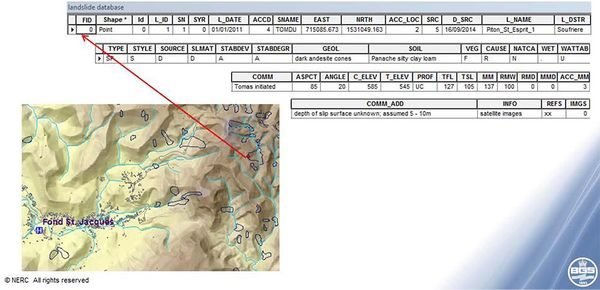
References
- ↑ CDB & CDERA (2006). Final Project Report: Development of landslide hazard maps for St Lucia and Grenada. The Caribbean Development Bank (CDB) and the Caribbean Emergency Response Agency (CDERA). February 2006, 53p
- ↑ ECLAC Report (2011). Saint Lucia: Macro socio-economic and environmental assessment of the damage and losses caused by Hurricane Tomas: a geo-environmental disaster. Towards Resilience. Economic Commission for Latin America and the Caribbean. 183pp.
- ↑ Van Westen C J (2014). Preliminary Assessment Report: CHARIM Caribbean Handbook on Risk Information management. ITC, University of Twente.
- ↑ Holcombe, E and Anderson, M. (2010). Tackling landslide risk: Helping land use policy to reflect unplanned housing realities in the Eastern Caribbean. Land Use Policy, 27, 798–800.
- ↑ Anderson, M and Holcombe, E. (2013). Community-Based Landslide Risk Reduction: Managing Disasters in Small Steps. The World Bank, Washington DC, 447p.
- ↑ Foster, C, Pennington, C V L, Culshaw, M G and Lawrie, K. (2012). The national landslide database of Great Britain: development, evolution and applications. Environmental Earth Sciences, 66(3), 941–953.
- ↑ Gibson, A D, Culshaw, M G, Dashwood, C and Pennington, C V L. (2013). Landslide management in the UK—the problem of managing hazards in a ‘low-risk’ environment. Landslides, 10(5), 599–610.
- ↑ Gibson, A D, Culshaw, M G, Dashwood, Cand Pennington, C V L. (2013). Landslide management in the UK—the problem of managing hazards in a ‘low-risk’ environment. Landslides, 10(5), 599–610.
- ↑ Foster, C, Pennington, C V L, Culshaw, M G and Lawrie, K. (2012). The national landslide database of Great Britain: development, evolution and applications. Environmental Earth Sciences, 66(3), 941–953.