OR/15/047 Resilience to future climate and abstraction
MacDonald A M, Bonsor H C, Taylor R, Shamsudduha M, Burgess W G, Ahmed K M, Mukherjee A, Zahid A, Lapworth D, Gopal K, Rao M S, Moench M, Bricker S H, Yadav S K, Satyal Y, Smith L, Dixit A, Bell R, van Steenbergen F, Basharat M, Gohar M S, Tucker J, Calow R C and Maurice L. 2015. Groundwater resources in the Indo‐Gangetic Basin: resilience to climate change and abstraction. British Geological Survey Internal Report, OR/15/047. |
Reliably forecasting the future nature of the Indian monsoon is proving difficult, with many Global Climate Models disagreeing on the impact of future emissions scenarios on both the timing and magnitude of rainfall (Gosht et al. 2012). However, progress is being made by the climate modelling community, and some of the key processes driving climate variability are being identified, uncovered and modelled (Turner et al. 2012[1]). From the perspective of groundwater, we need to understand how changes in the climate will impact on both abstraction and recharge. Looking at the issue from this perspective, there are some common elements of forecasts that could impact groundwater. There is a likelihood of increased storminess, with higher intensity rainfall as the energy in the climate system increases (IPCC 2013[2]; Jiménez‐Cisneros et al. 2014[3]). The timing of the start of the monsoon may also change. Temperature is highly likely to increase markedly, although this will be moderated in the basin by local effects of irrigation and evapotranspiration (Harding et al. 2013[4]). The increasing unreliability of rainfall and higher temperature are likely to exacerbate the demand for groundwater helping to sustain the rapid expansion of groundwater use (currently estimated at 2–5 km3 per year).
Groundwater recharge in the basin is controlled by: rainfall recharge (where the forecast increase in intensity of rainfall may lead to increased recharge, Taylor et al. 2013[5]); and leakage from canals and rivers. Future estimates of river flow are only as reliable as the future estimates of precipitation, which as discussed above are highly uncertain. For the Ganges, glacier melt comprises less than 10% of flow (Immerzeel et al. 2010[6]), much of which occurs during the monsoon period; therefore it is likely that future changes in glacier melt will not be a controlling influence on groundwater recharge. The situation in the Indus is different, with a much higher proportion of flow related to glacier melt. In the medium term this is likely to lead to higher river flows as the rate of melting of the glaciers increases (Jiménez‐Cisneros et al. 2014[3]). In the long term, when the contribution from glacial melt will reduce, it is postulated that increases in precipitation and snowmelt will compensate (Immerzeel et al. 2010[6]), although the uncertainty in future precipitation forecasting reduces the reliability of such predictions. A greater and more tangible risk to groundwater recharge are programmes to line tertiary canals and limit localised leakage and therefore limit recharge.
With such uncertainty about future precipitation and a likelihood of continued annual increases in abstraction (given the continuation of the drivers and incentives, such as energy subsidies, leading to groundwater abstraction) the resilience of the groundwater systems to change is a useful lens through which to examine the groundwater resource. Groundwater resilience to change is governed by the volumes of freshwater, the permeability of the aquifer system and the likely long term recharge (Foster and MacDonald 2014[7]).
Groundwater storage volumes and trends
The usable groundwater resource in the IGB is a function of the depth of the aquifer, the specific yield of the sediments (a measure of the drainable groundwater, related to the porosity) and the salinity of the groundwater. For this analysis we have limited the depth of the aquifer to 200 m, partly because so little information on aquifer properties or salinity is available below that depth, and also because so few boreholes currently abstract water from below 200 m except in the Bengal Basin.
Overall groundwater storage in the top 200 m of the IGB alluvial aquifer system is estimated at approximately 30 000 km3 ± 10 000 km3. For comparison, the estimated annual surface water flow from the Indus and Ganges‐Brahmaputra‐Meghna is approximately 1100 km3 (FAO Aquastat 2014[8]). The groundwater storage comprises 11 800 km3 of high quality fresh water with TDS <500 mg/L, 11 500 km3 of water with TDS 500–1000 mg/L, 3000 km3 with TDS 1000–2500 mg/L and 3300 km3 with water of the poorest quality with TDS >2500 mg/L. Groundwater abstraction comprises 205 km3 across the basin (in 2010), with much of it being abstracted from the freshwater areas: 159 km3 from storage with TDS <1000 mg/L, 32 km3 from TDS 1000–2500 mg/L and 13 km3 from TDS >2500 mg/L. Therefore, this large store of groundwater offers a significant buffer against short term changes in climate, with several decades of abstraction within the aquifer, even if recharge were to cease. However, declining groundwater levels can have a devastating impact on ecosystems, river flows and users of shallow groundwater, many of whom will not be able to access deeper groundwater.
Figure 18 shows the estimated current annual change in groundwater storage across the basin, estimated from the groundwater level map developed for the basin as part of this study. A complex picture of groundwater storage changes emerges governed both by the abstraction and the recharge. Highest depletion of storage is occurring in parts of the Indian Punjab, Haryana and Punjab Region in Pakistan where annual mass change of >100 mm equivalent of groundwater is occurring. If such trends of depletion continued, the groundwater resources in the upper 200 m in North West India and parts of the Punjab in Pakistan could be depleted to less than 50 % of their volume within 50 years. Similar trends are not observed in northern Bangladesh despite high abstraction, due to much greater natural recharge.
Groundwater accumulation also occurs — most notably in the Sindh, where groundwater has been accumulating at a rate of >10 mm per year. This is reflected in the water‐logging and corresponding salinity problems experienced in the Sindh. Groundwater accumulation also occurs across other parts of the basin — often close to where rapid depletion also occurs. This is a consequence of canal irrigation, where high leakage can cause rising groundwater levels and water‐logging within one part of the canal command, and rapid depletion further downstream.
Across the entire basin, the annual change in groundwater storage is estimated at approximately 10 km3. As discussed above, this integrates much complexity and variability within the IGB alluvial aquifer system, with some areas depleting and others accumulating. This compares well with the estimate of groundwater depletion using GRACE from Rodell et al. (2009)[9] who estimated 18 km3 depletion per year for the states of Rajasthan, Punjab and Haryana, with the majority of the depletion occurring in Rajasthan. Interpretation of GRACE data by Tiwari et al. (2009)[10] inferred a much higher rate of depletion (approximately 50 km3 per annum); however this work again had much of the depletion occurring outside the IGB alluvial aquifer system (e.g. within the poorer aquifer systems of Rajasthan) where recharge is negligible, and also indicated significant depletion in Bangladesh which was difficult to reconcile with observations (Shamsudduha et al. 2012[11]). More recent work on the GRACE data by Chen et al (2014)[12] suggests average depletion of 20 km3 per year across the region (again with significant depletion outside of the IGB alluvial aquifer system), with several years actually showing net accumulation. Using GRACE within this region to infer groundwater storage change is challenging: for example accounting for the significant changes in mass balance from ongoing tectonic activity, erosion and sedimentation; the large surface water flows within the river systems, the changes in moisture in the atmosphere, and the change in mass from snow and ice melt. Constraining and disaggregating the bulk estimates of mass change from GRACE to give groundwater storage changes required additional information and data. It is hoped that the groundwater storage changes developed here from analysis of the best available regional observational groundwater level data and specific yield information will be a useful tool to the further development and interpretation of GRACE data in the region.
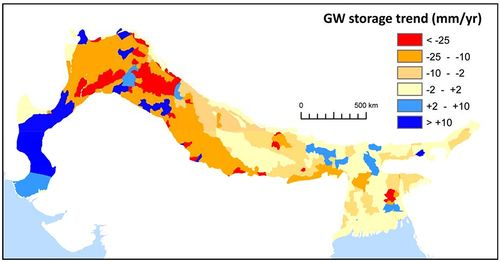
Groundwater recharge
As discussed earlier, groundwater recharge for the IGB alluvial aquifer is difficult to estimate given the combination of factors controlling it, from rainfall volumes and intensities to canal leakage in some areas, irrigation returns, river inundation and even abstraction. To help map out changes in groundwater recharge, we have estimated net groundwater recharge across the basin, calculated from the difference between the annual abstraction and the estimated water mass storage changes. The net recharge is therefore the average annual water being added to the system (or a reduction in natural discharge to rivers) to stop groundwater being depleted even more rapidly. The maps of both the estimated abstraction and water mass change are subject to uncertainty, although we have been careful to use the best possible available field data, triangulated and cross checked with other sources where available. Overall, given an annual abstraction of >200 km3 and an estimated annual depletion in storage of 10 km3, net recharge to the basin is high. However this masks the considerable variability.
Figure 19 shows the net recharge estimated for the basin. The map indicates substantial net groundwater recharge in areas of high irrigation suggesting that abstraction is increasing net recharge by reducing natural discharges to rivers, creating storage space within the aquifer and also returning some abstracted groundwater as recharge. So for example, net groundwater recharge has been significantly enhanced in the Indian States of Punjab and Haryana, in parts of the Punjab region of Pakistan and also in northern Bangladesh. There are likely to be different mechanisms for increasing net groundwater recharge in each case. In Northern Indian Punjab (Upper Indus and Upper–Mid Ganges typology), high rainfall recharge occurs and recycling of pumped groundwater irrigation water (see Box 4); while in the southern Indian Punjab and Pakistan (Middle Indus and Upper Ganges typology), the net groundwater recharge can only be accounted for if we account for the large volumes of canal leakage. For example, given an estimated annual flow in the canal systems of the Indus of 120 km3 and an estimated leakage to groundwater of 50%, then recharge of 60 km3 per year could be occurring. Estimating canal flows in the Ganges canal systems is more difficult since data are not publically available; however, given a design capacity of approximately 300 km3 and similar leakage to the Indus systems, recharge could be highly significant. In Bangladesh, groundwater levels are shallow, and increased abstraction has been shown to create space within the aquifer for additional recharge during the monsoon (Shamsudduha et al. 2012[11]).
A caveat must be added to the above discussion, in that it is reliant on the quality of the existing groundwater level data for the basin. Although every effort has been made to QA and check the datasets used, there is still an absence of reliable representative groundwater level data across the IGB alluvial aquifer system. Only with improved groundwater level monitoring networks can more confident analysis be undertaken; as discussed above, it is unwise to rely on unconstrained large scale remotely sensed gravity data.
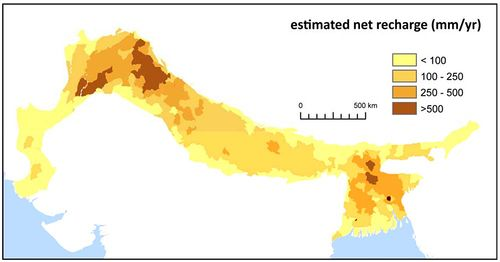
Box 4 — Case study: Groundwater response to abstraction and recharge processes in Northern India Punjab in northwest India is a key area for India’s food production (e.g. rice, wheat and sugar) and both groundwater and surface water are intensely used for irrigation. Groundwater levels have been observed to be falling up to 80 cm per year in some places and pre monsoon groundwater levels can be >20 mbgl. As a consequence deeper tubewells are now being drilled to abstract groundwater from depths of 150 m, augmenting abstraction from shallower tubewells. Understanding groundwater recharge processes and the connections between shallow and deeper groundwater will be vital to help manage the groundwater abstraction and reduce the impacts of unsustainable abstraction. Case study objectives: A focussed hydrogeological study was carried out in the Bist‐Doab area of northern Punjab, northwest India, to gather new evidence on recharge processes, groundwater quality, groundwater residence times, and the connectivity of shallow and deep groundwater. The study analysed 20‐year records of groundwater level variations and undertook repeated sampling of shallow and deep piezometers using a suite of environmental tracers — including groundwater age tracers CFCs and SF6, stable isotopes and noble gases (recharge source tracers). Nineteen paired shallow (8–50 mbgl) and deep (76–160 mbgl) tubewells were sampled both pre and post monsoon to provide new evidence on groundwater recharge and flow processes across a range of hydrological settings. Detailed results from the study are given in Lapworth et al (2015)[13]. Key results: Groundwater levels are not declining throughout the study area: but mostly at the end of the canal command areas, with more stable groundwater levels throughout other parts of the catchment. The widespread occurrence of modern tracers in deep groundwater (>60% of sites had modern fractions >0.1) suggests that there is low aquifer anisotropy here and that deep aquifers are recharged by a significant component of modern (<75 years old) recharge via vertical leakage. Stable isotope and noble gas results at all depths indicated modern meteoric conditions, with little evidence of widespread recharge from canal leakage in this area. Groundwater recharge is dominated by rainfall in this northern part of the Punjab.
|
So what of recharge to the IGB alluvial aquifer in the future? The climate forecasts do not suggest widespread and rapid reduction in precipitation volumes across the basin. Given the evidence that rainfall recharge can occur even where precipitation is low (<350 mm) and may be linked to intensity of rainfall events, significant rainfall recharge is likely to carry on within the bounds forecast for future climate variability. River flow is also not forecast to be severely impacted by climate change, or even glacier melting. However, river flow, particularly in the Indus, has been significantly impacted by diversions for irrigation, with severe impacts for the quality of water particularly in the Sindh. Canal leakage forms an important component of groundwater recharge, particularly in drier areas. Programmes to line tertiary canals, if not accompanied by a reduction in groundwater abstraction could have a much greater impact on groundwater recharge than any direct influence from climate change. Another area to focus on is returns from irrigation to the groundwater. Using more efficient irrigation methods may reduce this loss, and therefore reduce net groundwater recharge.
Degradation
The degradation of water quality is arguably the greatest threat to the IGB aquifer. Within the Indus Basin and parts of the Ganges, water management practices have led to significant degradation in groundwater quality, and continue to do so. Three of the most pressing issues are: increased salinization of the groundwater resources, contamination from agriculture and urban centres, and the mobilisation of arsenic and other naturally occurring contaminants. In this section we discuss salinization and agricultural contamination, arsenic and urban issues are considered separately below.
Irrigation and abstraction has led to considerable salinization of groundwater in the Mid Indus, Upper Ganges typology, and the Lower Indus groundwater typology — Figure 20. Canal leakage and irrigation returns, although useful for groundwater recharge, have also degraded the quality of the groundwater through water‐logging and subsequent phreatic salinization. It is possible that increased groundwater abstraction has been reducing this trend by lowering groundwater levels, however, high abstraction has also mobilised older, deeper saline water into shallow depths. This is evident in Mid Indus/Upper Ganges typology where high abstraction is being accompanied by increasingly saline water. In the Indus, the reduction of outflow of water at the Kotri Barrage means that there is little or no natural outflow from the basin. Therefore, the salts generated from weathering of the rocks in the Himalaya are all retained within the basin, leading inevitably to an increase in salt concentration in the groundwater. Within the lower Indus, much of the groundwater is already saline, and the shallow freshwater lenses are highly vulnerable to abstraction.
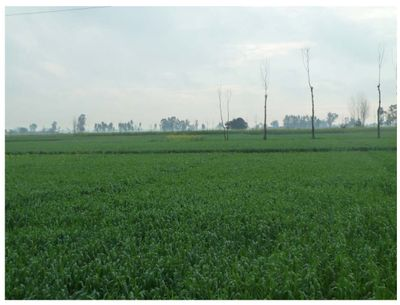
Intense groundwater abstraction is also accompanied by increases in the use of fertilizer, pesticide and herbicides. Elevated nitrate concentrations are found within the Punjab (Lapworth et al. 2014[14]), and have been drawn down to >100 m depth by deep pumping. Elevated nitrate concentrations are also likely to be associated with the presence of other agriculturally derived contaminants. Recycling of groundwater through abstraction, irrigation and irrigation returns has also been shown to intensify this process (Ó Dochartaigh et al, 2010[15]), with increasing concentrations of solutes and contaminants within irrigation returns derived from groundwater. Routinely monitoring groundwater quality enables trends in degrading quality to be identified and strategies developed to try to mitigate the problem.
Resilience of deep groundwater abstraction (in the Bengal Basin) against contamination by arsenic and salinity
The potential vulnerability of deep wells to contamination by arsenic and salinity drawn down over time from shallow and intermediate levels is critical to the security of water supply in Bangladesh (Burgess et al. 2010[16]). Michael and Voss (2008)[17] have concluded from a regional modelling study of the Bengal basin that deep groundwater “could provide arsenic‐safe drinking water to >90% of the arsenic‐impacted region over a 1000‐year timescale, if its utilization is limited to domestic supply”. In a similar region a modelling study of SE Bangladesh, UCL (2013) have concluded that “deep groundwater abstraction for public water supply in southern Bangladesh is in general secure against ingress of arsenic for at least 100 years”.
Locally, deep groundwater is vulnerable to arsenic or salinity even under present pumping patterns, but these impacts are manageable through a programme of monitoring that could provide many years advance warning of impending problems. Despite concern for sustainability of the deep groundwater resource, there is little empirical evidence for an adverse impact on quality or water levels that can be attributed to deep groundwater pumping. In the Pakistan Punjab, there is evidence of upconing of deeper saline groundwater from abstraction. Across the basin, arsenic exceeds 10 μg/L in 18% of deep wells (Burgess et al. 2010[16]), but whether this is a result of breached well casings or hydrological response to pumping remains uncertain. Empirical re‐appraisal of 46 deep wells in south‐central Bangladesh (Ravenscroft et al., 2013[18]) shows groundwater composition at >150 m depth has remained largely unchanged for the 13 years between 1998 and 2011 and with no deterioration inferred over the operating lifetimes of the deep tubewells concerned, between 20 and 43 years.
Therefore, the deeper groundwater resources in the Bengal basin have a high general resilience to change induced by climate or pumping: they are largely divorced from the modern climate, with negligible modern recharge, and at a regional level there appears little evidence of widespread changes in arsenic concentrations. However, at a highly localised level, around individual wells, the heads induced by pumping, and disruption to the aquifer system during drilling can lead to localised contamination at depth/localised contamination pathways. Therefore routine monitoring both of the regional aquifer system and individual pumping wells is paramount.
High abstraction from cities
Groundwater from the IGB alluvial aquifer system sustains many of the region’s cities, notably, New Delhi, Dhaka and Lahore. Although urban groundwater has not been a focus of this study, given that the total volumes abstracted are small compared to irrigation, and urban groundwater is a highly complex issue, the resilience of the water supplies to these cities is of major concern. The high density of the abstraction (e.g. for Dhaka an estimated 0.8 km3 within 300 km2 DWASA 2012) and the mixture of private and public water supplies, means groundwater is locally becoming highly depleted in these cities with groundwater levels falling rapidly (>100 m depth in some locations) and bringing with it a set of issues, such as subsidence, declining yields, increased costs (Morris et al. 2003[19]) — Figure 21. In tandem with the high abstraction is pervasive, and widespread, contamination of groundwater from both domestic water (sewerage) and industrial waste (Foster and Choudhary 2009[20]). The stratification of the aquifers protects some of the deeper groundwater from contamination, but shallow groundwater, often used for private water supply, can by highly contaminated. Therefore, continued good quality groundwater supply in the largest cities will be difficult to sustain in the long term, without developing large protected wells fields outside of the urban areas and building groundwater protection into land use planning. A systematic assessment of the scale and significance of existing groundwater pollution in city regions is also required, together with a robust evaluations of private water well use and the role of institutions in relation to urban groundwater use and protection (Foster and Choudhary 2009[20]; Foster et al. 2010[21]).
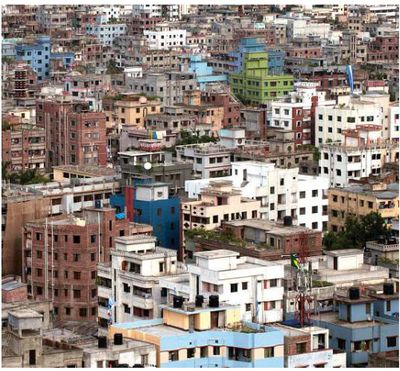
References
- ↑ Turner, A G Annamalai H. 2012. Climate change and the South Asian summer monsoon. Nature Climate Change 2 587–595.
- ↑ IPCC. 2013. Climate Change 2013: The Physical Science Basis, pp 1535.
- ↑ Jump up to: 3.0 3.1 Jiménez‐Cisneros, B E, Oki, T, Arnell, N W, Benito, G, Cogley, J G, Döll, P, Jiang, T and Mwakalila, S S. 2014. Freshwater resources. In: Climate Change 2014: Impacts, Adaptation, and Vulnerability. Part A: Global and Sectoral Aspects. Contribution of Working Group II to the Fifth Assessment Report of the Intergovernmental Panel on Climate Change [Field, C B, Barros, V R, Dokken, D J, Mach, K J, Mastrandrea, M D, Bilir, T E, Chatterjee, M, Ebi, K L, Estrada, Y O, Genova, R C, Girma, B, Kissel, E S, Levy, A N, MacCracken, S, Mastrandrea, P R and White, L L. (eds.)]. Cambridge University Press, Cambridge, United Kingdom and New York, NY, USA, pp. 229–269.
- ↑ Harding R J, Blyth E M, Tuinenburg O A and Wiltshire A. 2013. Land atmosphere feedbacks and their role in the water resources of the Ganges basin, Science of the Total Environment, 468–469, S85‐92.
- ↑ Taylor R. et al. 2013. Groundwater and climate change, Nature Climate Change 3; 322–329, doi: 10.1038/nclimate1744
- ↑ Jump up to: 6.0 6.1 Immerzeel W W, Pellicciotti F and Bierkens M F P. 2013. Rising river flows throughout the twenty‐first centrury in two Himalayan glacierized watersheds, Nature Geoscience, Letters, doi: 10.1038/NGEO1896
- ↑ Foster S and MacDonald A M. 2014. The 'water security' dialogue: why it needs to be better informed about groundwater. Hydrogeology Journal, 22; 7, 1489–1492.
- ↑ FAO AQUASTAT 2014. Food and agriculture organization of the United Nations (Accessed Feb 2015)
- ↑ Rodell M, Velicogna I and Famiglietti J S. 2009. Satellite‐based estimates of groundwater depletion in India, Nature, 460; 999–1002.
- ↑ Tiwari V M, Whar J and Swenson S. 2009. Dwindling groundwater resources in northern India, from satellite gravity observations, Geophysical research Letters, 36; L18401, doi: 10.1029/2009GL039401.
- ↑ Jump up to: 11.0 11.1 Shamsudduha M, Taylor R G & Longuevergne L. 2012. Monitoring groundwater storage changes in the highly seasonal humid tropics: validation of GRACE measurements in the Bengal Basin. Water Resources Research, 48; WO2508, doi: 10.1029/WR010993
- ↑ Chen J, Li J, Zhang Z and Ni S. 2014. Long‐term variations in Northwest India from Satellite gravity measurements, Global and Planetary Change, 116; 130–138.
- ↑ Lapworth D J, MacDonald A M, Krishan G, Rao M S, Gooddy D C and Darling W G. 2015. Groundwater recharge and age‐depth profiles of intensively exploited groundwater resources in northwest India. Geophysical Research Letters, Submitted.
- ↑ Lapworth D J, Gopal K, Rao M S, MacDonald A M. 2014. Intensive Groundwater Exploitation in the Punjab – an evaluation of resource and quality trends, British Geological Survey Open Report, Groundwater Science Programme, OR/14/068.
- ↑ O Dochartaigh B E, MacDonald A M, Darling W G, Hughes A G, Li J X and Shi L A. 2010. Determining groundwater degradation from irrigation in desert‐marginal northern China, Hydrogeology Journal, 18; 1939–1952.
- ↑ Jump up to: 16.0 16.1 Burgess W G, Hoque M A ,Michael H A, Voss C I, Breit G N and Ahmed K M 2010 Vulnerability of deep groundwater in the Bengal Aquifer System to contamination by arsenic. Nature Geoscience 3: 83–87
- ↑ Michael H A and Voss C I 2008. Evaluation of the sustainability of deep groundwater as an arsenic — safe resource in the Bengal Basin. PNAS 105 (25) 8531‐8536
- ↑ Ravenscroft P, McArthur J M and Hoque M A. 2013. Stable groundwater quality in deep aquifers of Southern Bangladesh: the case against sustainable abstraction, Science of the Total Environment 454‐456: 627–638
- ↑ Morris, B L, Lawrence, A R, Chilton, P J, Adams, B, Calow, R and Klinck, B A. 2003). Groundwater and its Susceptibility to Degradation: A Global Assessment of the Problems and Options for Management. Early Warning and Assessment Report Series, RS, 03‐3. United Nations Environment Programme, Nairobi.
- ↑ Jump up to: 20.0 20.1 Foster S and Chaudhary NK. 2009. Lucknow City — India: Groundwater Resource Use and Strategic Planning Needs, GW‐MATE Case Profile Collection, in Sustainable Groundwater Management: Lessons from Practice, World Bank, pp 8.
- ↑ Foster S, van Steenbergen F, Zuleta J and Garduno H. 2010. Conjuctive use of groundwater and surface water — from spontaneous coping strategy to adaptive resource management. GW‐MATE Strategic Overview Series 2: World Bank, Washington DC, pp 26.